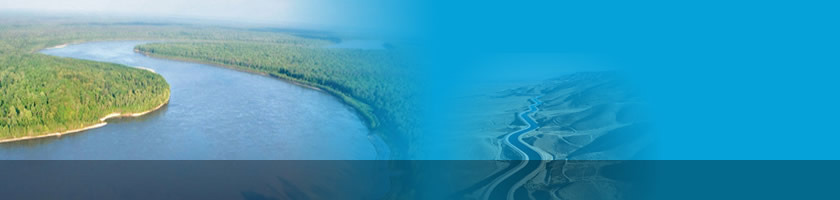







Vol.5 , No. 3, Publication Date: Jun. 28, 2018, Page: 39-55
[1] | Philip Chi-Wah Cheung, Department of Chemical Engineering, Imperial College, London, United Kingdom. |
[2] | Daryl Robert Williams, Department of Chemical Engineering, Imperial College, London, United Kingdom. |
[3] | Donald Wilfrid Kirk, Department of Chemical Engineering, University of Toronto, Toronto, Canada. |
[4] | James Barker, Department of Chemical and Pharmaceutical Sciences, Kingston University, Kingston-on-Thames, United Kingdom. |
In this article experimentation to establish the feasibility of a chemical reaction between free radical chlorine dioxide ClO2 and chelated complexes of Co2+, Ni2+ and Cu2+ with citric acid (C6H8O7) and ethylenediaminetetraacetic acid (EDTA, C10H16N2O8) as ligands, leading to the stoichiometric recovery of the metallic ions, is described. The procedure is one of empirical observation and discovery in the laboratory. This novel concept of the chemical-induced separation process predicated upon its ability to extract and precipitate metallic ions as insoluble metal oxides and oxyhydroxides, but not as hydroxides which are epitomized by the green hydrophilic gel Ni(OH)2 and extremely difficult to dewater. Chelation technology has many applications in industry and the wastewaters from these operations also contain a myriad of non-complexing agents such as brighteners used in electroplating. This work explores the option of in-house recovery of valuable resources such as transition metals, recognizing that the most formidable challenge is the separation of metallic ions from the organic ligands that protect them from precipitation in alkaline environments. Simulated wastewaters are used to study this reaction, free from the interference of proprietary additives and imposed operational conditions of the commercial sectors. The near 99% recovery of cobalt, nickel and copper ions is successful. This creates the possibility of scaling up from laboratory glassware to larger reactor vessels such as a semi-batch reactor in a small pilot-plant facility. This present work is not about the production of potable water, but much expertise has been accumulated pertaining to the efficacy of chlorine dioxide as a disinfectant and some of the relevant literature deserves attention here. The “future work” section discusses process development by way of a pilot plant investigation. It must be emphasized that this technology is not developed to be applied in public sewage treatment and no such inference should be made. (75 references from 1963 to 2018).
Keywords
Chlorine Dioxide, Cobalt, Nickel, Copper, Citrate, EDTA, Recycling
Reference
[01] | Payne J. G. (2017). Chapter 6: “Industry”. United Nations World Water Development Report: Wastewater, the Untapped Resource. UNESCO (Paris, France), March 22, 2017, pp. 58-77. |
[02] | Cheung P., Williams D., Kirk D. (2018). Letter to the Editors: Comments on “Encapsulation in ceramic material of the metals Cr, Ni, and Cu contained in galvanic sludge via the solidification and stabilization method. Journal of Environmental Chemical Engineering, 5 (2017), pp. 3834-3843”. Article in Press, doi: 10.1016/j.jece.2017.10.003. |
[03] | Oviedo C., Rodríguez J. (2003). EDTA: The chelating agent under environmental scrutiny. Química Nova, 26 (6), 2003, pp. 901-905. |
[04] | Smith S. (2001). Pollutants in urban wastewater and sewage sludge. Final report to the Directorate General of the Environment (DG11), European Commission, Brussels, 2001. (The report is published by Imperial College Consultants, 2001). |
[05] | Kari F. G., Giger W. (1996). Speciation and Fate of Ethylenediaminetetraacetate (EDTA) in Municipal Wastewater Treatment. Water Research, 1996, 30 (1), pp. 124-134. |
[06] | Bedsworth W. W., Sedlak, D. L. (1999). Sources and Environmental Fate of Strongly Complexed Nickel in Estuarine Waters: the Role of Ethylenediamine-tetraacetate. Environmental Science and Technology, 1999, 33 (6), pp. 926-931. |
[07] | Cheung P., Williams D. R. (2015). Separation of Transition Metals and Chelated Complexes in Wastewaters”, Environmental Progress and Sustainable Energy, 34 (3), 2015, pp. 761-783. |
[08] | Aquarden Technolgies (2017). Are you ready to phase out Chromium-6? Aquarden Technologies ApS, 2017. Available at: http://aquarden.com/wp-content/uploads/2017/03/White-paper-Chromium-6.pdf. (Accessed on May 10, 2018). |
[09] | Mallory G. O., Hajdu J. B. (1990). Electroless plating: fundamentals and principles (bk). American Electroplaters and Surface Finishers Society, Orlando, Florida, USA, 1990. |
[10] | Sayed S. Abd El Rehim, Saad M. Abd El Wahaab, Magdy A. M. Ibrahim, Marwan M. Dankeria. (1999). Electroplating of cobalt from aqueous citrate baths. Journal of Chemical Technology and Biotechnology, 73 (4), 1998, pp. 369-376. |
[11] | Haran B. S., Polov B. N., White R. E. (1998). Studies on Electroless Cobalt Coatings for Microencapsulation of Hydrogen Storage Alloys. Journal of the Electrochemical Society, 145 (9), 1998, pp. 3000-3007. |
[12] | Doi T., Mizumoto K. (2004). Effect of bath pH on nickel citrate electroplating bath. Metal Finishing, 102 (6), 2004, pp. 104-111. |
[13] | Field T. B., McCourt J. L., McBryde W. A. E. (1974). Composition and stability of iron and copper citrate complexes in aqueous solution. Canadian Journal of Chemistry, 52, 1974, pp. 3119 – 3124. |
[14] | Jamal Amar, Sameer Al-Khawaja. (2014). Nickel coated on beech wood by electroless nickel coating: effects of complexing agent concentrations on nickel film properties. Journal of Composite Interfaces, 21 (8), 2014, doi.org/10.1080/15685543.2014.914390. |
[15] | Mizumoto S., Nawafune H., Kojima T., Haga M., Shiraishi M. (1991). Electroplating of copper from EDTA complex bath and miniscale throwing power. Circuit Technology, 6 (1), 1991, pp. 1-7. |
[16] | McCoy E. H. (1983). Alkaline plating baths and electroplating process. U.S. Patent 4,389,286A. |
[17] | Szöke S., Phtzay G., Weiser L. (2005). Cobalt (III) EDTA complex removal from aqueous alkaline borate solutions by nanofiltration. Desalination, 175 (2), 2005, pp. 179-195. |
[18] | Martins J. I., Nunes M. C., Tavares P. B. (2016). Copper electroless plating in presence of cobalt ions using hypophosphite as reductants. Corrosão E Protecção de Materiais, 35 (1), 2016, pp. 6-14. |
[19] | Toda A., Chivavibul P., Enoki M. (2013). Effects of plating conditions on electroless Ni-Co-P coating prepared from lactate-citrate-ammonia solution. Materials Transactions, 54 (3), 2013, pp. 337-343. |
[20] | Shipley C. R. Jr., Shipley L., Gulla M., Dutkewych O. B. (1971). Electroless Copper Plating. U.S. Patent 3,615,735. |
[21] | Gilles M. K., Polak M. L., Lineberger W. C. (1992). Photoelectron Spectroscopy of the Halogen Oxide Anions FO-, CIO-, BrO-, IO-, OCIO-, and OIO-. Journal of Chemical Physics, 1992, 96 (11), pp. 8012-8020. |
[22] | Rehr A., Jansen, M. (1992). Investigations on Solid Chlorine Dioxide: Temperature-Dependent Crystal Structure, IR Spectrum, and Magnetic Susceptibility. Inorganic Chemistry, 1992, 31 (23), pp. 4740-4742. |
[23] | Holleman A. F., Wiberg E. (2001). Oxides and Fluoroxides of the Halogens. Chap. 5 in Inorganic Chemistry (bk), Academic Press, 2001, p. 461. |
[24] | Cotton F. A., Wilkinson G., Gaus P. L. (1995). Diagram of Chlorine Dioxide Dimer. (1995). Chapter 20, The Halogens: Fluorine, Chlorine, Bromine, Iodine and Astatine, Basic Inorganic Chemistry (bk), 3rd ed., John Wiley, 1995, p. 470. |
[25] | Housecroft C. E., Sharpe A. G. (2008). Oxides and Oxofluorides of Chlorine, Bromine and Iodine. Chap. 17 in Inorganic Chemistry (bk), 3rd ed., Prentice Hall, 2008, pp. 551. |
[26] | U.S. Environmental Protection Agency (1999). U.S. EPA Guidance Material, Chapter 4: Chlorine Dioxide. In: Alternative Disinfectants and Oxidants (Guidance Manual 815-R-99-014), page 4.1. |
[27] | Stevens A. A. (1982). Reaction products of chlorine dioxide. Environmental Health Perspectives, 46, 1982, pp. 101-110. |
[28] | Werdehoff K. S., Singer P. C. (1987). Chlorine Dioxide Effects on THMFP, TOXFP and the Formation of Inorganic By-Products. Journal of the American Water Works Association, 1987, 79 (9), pp. 107-113. |
[29] | World Health Organization (2018). Water Sanitation Hygiene: Chemical hazards in drinking-water: Chloramines (monochloramine, dichloramine, trichloramine). Available at: http://www.who.int/water_sanitation_health/water-quality/guidelines/chemicals/chloramine/en/ Accessed: (May 10, 2018). |
[30] | Suh D. H., Abdel-Rahman M. S. (1985). Mechanism of Chloroform Formation by Chlorine and Its Inhibition by Chlorine Dioxide. Toxicological Sciences, 1985, 5 (2), pp. 305-313. |
[31] | Wang Y. L., Liu H. J., Liu G. G., Xie Y. H., Liu X. Y. (2015). Kinetics for diclofenac degradation by chlorine dioxide in aqueous media: Influences of natural organic matter additives. Journal of the Taiwan Institute of Chemical Engineers, 56 (November 2015), pp. 131-137. |
[32] | Van Haute S., Tryland I., Escudero C., Vanneste M., Sampers I. (2017). Chlorine dioxide as water disinfectant during fresh-cut iceberg lettuce washing: Disinfectant demand, disinfection efficiency, and chlorite formation. LWT - Food Science and Technology, 75, 2017, pp. 301-304. |
[33] | Yunho Lee, Urs von Gunten. (2010). Oxidative transformation of micropollutants during municipal wastewater treatment: Comparison of kinetic aspects of selective (chlorine, chlorine dioxide, ferrate VI, and ozone) and non-selective oxidants (hydroxyl radical). Water research 44 (2), 2010, pp. 555-566. |
[34] | OxyChem (2009). Technical Services Department. Laboratory Preparations of Chlorine Dioxide Solutions. Technical Data Sheet 600-102, Occidental Chemical Corporation, Dallas, Texas, USA, March, 2009. Available at: http://www.oxy.com/OurBusinesses/Chemicals/Products/Documents/SodiumChlorite/Laboratory%20Preparations%20of%20Chlorine%20Dioxide%20Solutions.pdf. (Accessed: May 10, 2018). |
[35] | CRC Handbook of Chemistry & Physics (ed. Haynes W. M), 91st ed., 2010-2011, pp. 821. |
[36] | Gates D. J. (1989). Improvements in chlorine dioxide use: A two-step method for determining residual oxidants in the presence of other chlorine species in finished water. In: Advances in water analysis and treatment: Proceedings of the American Water Works Association Water Quality Technology Conference, November 13-17, 1988, St. Louis, MO. American Water Works Association, Denver, CO. pp. 689-703. |
[37] | Aieta E., Berg J. D. (1986). A Review of Chlorine Dioxide in Drinking Water Treatment. Journal of the American Water Works Association, 1986, 78 (6), pp. 62-72. |
[38] | Cheung P., Williams D. R. (2015). Separation of Transition Metals and Chelated Complexes in Wastewaters”, Environmental Progress and Sustainable Energy, 34 (3), 2015, pp. 761-783. |
[39] | Aramyan S. M., Moussavi M. (2017). Advances in Fenton and Fenton Based Oxidation Processes for Industrial Effluent Contaminants Control: A Review. International Journal of Environmental Science and Natural Resources, 2 (4), 2017, pp. 1-18. |
[40] | Lin Q, Pan H, Yao K, Pan Y, Long W. (2015). Competitive removal of Cu-EDTA and Ni-EDTA via microwave-enhanced Fenton oxidation with hydroxide precipitation. Water Science and Technology, 72 (7), 2015, pp. 1184-90. |
[41] | Zhao B, Zhu W., Mu T., Hu Z., Duan T. (2017). Electrochemical Oxidation of EDTA in Nuclear Wastewater Using Platinum Supported on Activated Carbon Fibers. International Journal of Environmental Research and Public Health, 14, 2017, pp. 1-18, doi: 10.3390/ijerph14070819. |
[42] | OxyChem (2009). Technical Services Department. Laboratory Preparations of Chlorine Dioxide Solutions. Technical Data Sheet 600-102, Occidental Chemical Corporation, Dallas, Texas, USA, March, 2009. Available at: http://www.oxy.com/OurBusinesses/Chemicals/Products/Documents/SodiumChlorite/Laboratory%20Preparations%20of%20Chlorine%20Dioxide%20Solutions.pdf. (Accessed: May 10, 2018) |
[43] | Engstrom J. (1992). Process for the production of chlorine dioxide. U.S. Patent 5154910A, published October 13, 1992. |
[44] | White G. C. (1992). The Handbook of Chlorination and Alternative Disinfectants, 3rd ed., Van Nostrad Reinhold, New York, 1992, pp. 984. |
[45] | Horiba Jobin Yvonne (2018). A Platform for the Future: Technical Manual on the Ultima 2C Series, Combination ICP-OES. Available at: http://photos.labwrench.com/equipmentManuals/7573-2798.pdf. (Accessed: May 10, 2018). |
[46] | Harris D. (2007). EDTA Titrations. Chap. 12 in Quantitative Chemical Analysis (bk), W. H. Freeman: New York, 2007, pp. 231, 233, 234. |
[47] | Widyarani D., Hariyadi H. R., Wulan D. R., Cahyaningsih S. (2016). Removal of nickel ion from electroplating wastewater using double chamber electrodeposition cell (DCEC) reactor partitioned with water hyacinth Eichhornia crassipes leaves. International Conference on Recent Trends in Physics 2016. Journal of Physics: IOP Conference Series 755 (2016) 011001 doi: 10.1088/1742-6596/755/1/011001. |
[48] | Brown C. J. (1987). Acid and metals recovery by Recoflo short bed ion exchange. Chapter 36 in Separation Processes in Hydrometullurgy (bk., editor G. A. Davies), Ellis Horwood Ltd., 1987, pp. 383. |
[49] | Tratnyek P. G., Hoigné J. (1994). Kinetics of reactions of chlorine dioxide (OCIO) in water-II. Quantitative structure-activity relationships for phenolic compounds. Water Research, 28 (1), pp. 57-66, 1994. |
[50] | Bruice, P. Y. (2004). Decarboxylation of 3-Oxocarboxylic Acids. Section 19.17, Chap. 19: Carbonyl Compounds III, in Organic Chemistry, 4th ed.; Prentice Hall, 2004, pp. 819. |
[51] | Rund J. V., Plane R. A. (1963). Catalysis of the decarboxylation of dimethyloxaloacetate by Manganese (11), Nickel (II1, and their complexes. Journal of the American Chemical Society, 86 (3), 1964, pp 367-371. |
[52] | Goldstein S., Meyerstein D. (1999). Comments on the Mechanism of the “Fenton-Like” Reaction. Accounts of Chemical Research, 32 (7), 1999, pp. 547-550. |
[53] | Stanbury D. M., Lednickyt L. A. (1983). Outer-Sphere Electron-Transfer Reactions Involving the Chlorite/Chlorine Dioxide Couple. Activation Barriers for Bent Triatomic Species. Jounral of the American Chemical Society, 105 (10), pp. 3098-3101. |
[54] | Burke I. (2007). Modern treatment methods of strong chelates in surface technology. Surface World Magazine, April 2007. Available at: http://pdfs.findtheneedle.co.uk/126875-3361.pdf. (Accessed: May 10, 2018). |
[55] | Mitsos A. (2018). Conceptual Design of Chemical Processes. Lecture notes, Process Systems Engineering, 2018, Aachener Verfahrenstechnik (RWTN-Aachen University), Aachen, Germany. |
[56] | Lee S. G. (Water Technologies Limited). (2000). Chlorine dioxide Technology systems. Available at: https://sunggyuleephd.com/clo2-2/. (Accessed: May 10, 2018). |
[57] | U.S. EPA. (1999). Chlorine Dioxide Generation Technology and Mythology. Chap. 4 in Alternative Disinfectants and Oxidants Guidance Manual, EPA 815-R-99-014, April 1999, pp. 4.1-4.39. Available at: https://www.discountpdh.com/course/disinfecting_the_water_using_combined_disinfectants/disinfecting_the_water_using_combined_disinfectants.pdf. (Accessed: May 10, 2018). |
[58] | Brown P. L., Ekberg C. (2016). Chapter 11, Hydrolysis of Metal Ions, Vol. 2 (bk), Wiley-VCH 2016. |
[59] | Tan J. J., Huang Y., Wu Z. Q., Chen Xi. (2017). Ion exchange resin on treatment of copper and nickel wastewater. IOP Conference Series: Earth and Environmental Science, 94, 2017, 012122. Available at: http://iopscience.iop.org/article/10.1088/1755-1315/94/1/012122/pdf. (Accessed: May 10, 2018). |
[60] | Collivignarelli M. C., Abbà A., Benigna I., Sorlini S., Torretta V. (2018). Overview of the main disinfection processes for wastewater and drinking water treatment plants. Sustainablity, 10 (86), 2018, pp. 1-21. |
[61] | Griese M. (1991). Pilot plant optimization of the chlorine dioxide treatment process for disinfection by-product reduction. U.S. Environmental Protection Agency, Washington D. C., EPA/600/A-94/192 (NTIS PB95125431), 1991. |
[62] | Littrell L., Romano S., Dorris R., Parra G., Gallarda M., Kuhn J. (2017). Chlorine dioxide treatment for disinfection byproduct reduction. Florida Water Resources Journal, November 2017, pp. 22-29. |
[63] | Holden, G. W., (2017). Chlorine dioxide preoxidation for DBP reduction. Journal of the American Water Works Association, 109, pp 36-43. |
[64] | Jiang J., Zhang X., Zhu X., Li Y. (2017). Removal of intermediate aromatic halogenated DBPs by activated carbon adsorption: a new approach to controlling halogenated DBPs in chlorinated drinking water. Environmental Science and Technology, 2017, 51 (6), pp 3435-3444. |
[65] | Lykins B. W., DeMarco J. (1983). Pilot plant study on the use of chlorine dioxide and granular activated carbon. Advances in Chemistry, 202, 1983, pp. 425-453. |
[66] | Dickinson E. (2018). Removal of contaminants during pilot-scale ozone, biological activated Carbon, and granular activated carbon for potable reuse. American Water Works Association, International Symposium on Potable Reuse, Austin, Texas, January 22 – 23, 2018. |
[67] | Shimizu Y., Ateia M., Yoshimura C. (2018). Natural organic matter undergoes different molecular sieving by adsorption on activated carbon and carbon nanotubes. Chemosphere, 203, 2018, pp. 345-352 (in progress). |
[68] | Rittmann D., Tenney J. (2000). Generating Chlorine Dioxide Gas: Chlorate vs. Chlorite. Water and Wastes Digest, December 28, 2000. Available at: http://www.wwdmag.com/water/generating-chlorine-dioxide-gaschlorate-vs-chlorite. (Accessed: May 10, 2018). |
[69] | World Health Organization (2000). Disinfectants and disinfectant by-products. Environmental Health Criteria 216, Chapter 2, pp. 27, Geneva, 2000. |
[70] | Sutton S. C. (2017). An Integrative Synthetic, Spectroscopic, and Computational Study of the Free Radical Chlorine Dioxide and its Interactions with Hydrogen Bonded Networks. (A thesis submitted to the faculty of The University of Mississippi in partial fulfillment of the requirements of the Sally McDonnell Barksdale Honors College) Available at: http://laser.chem.olemiss.edu/~hammergroup514/theses/Sutton_Thesis-finaldraft.pdf (Accessed: May 10, 2018). |
[71] | Alegre M. L. et. al., 7 authors. (2000). Kinetic study of the reactions of chlorine atoms and Cl2•- radical anions in aqueous solutions. 1. Reaction with Benzene. Journal of Physical Chemistry A, 104 (14), 2000, pp. 3117-3125. Available at: https://pubs.acs.org/doi/pdf/10.1021/jp9929768. (Accessed: May 10, 2018). |
[72] | Olah G. A., Comisarow M. B. (1968). Radical cations. I. Direct electron paramagnetic resonance observation of the chlorine molecule cation Cl2•+, a stable diatomic radical cation, in SbF5, FSO3H-SbF5, and HF-SbF5 solutions of ClF. Journal of the American Chemical Society, 1968, 90 (18), pp. 5033–5034. Available at: https://pubs.acs.org/doi/abs/10.1021/ja01020a052. (Accessed: May 10, 2018). |
[73] | Cacace F., de Petris G., Troiani A., Cooks R. G. (1999). Isomeric Cl2O2+ and Cl2O2- ions. Rapid Communications in Mass Spectrometry, 13 (19), 1999, pp. 1903-1906. |
[74] | Schecher W. D., McAvoy D. C. (1992). MINEQL+, A software environment for chemical equilibrium modeling. Computers, Environments and Urban Systems, 16 (1), 1992, pp. 65-76. |
[75] | Environmental Research Software (2015). Information sheet: What is MINEQL+? Available at: https://www.mineql.com/Overview.html. (Accessed: May 10, 2018). |